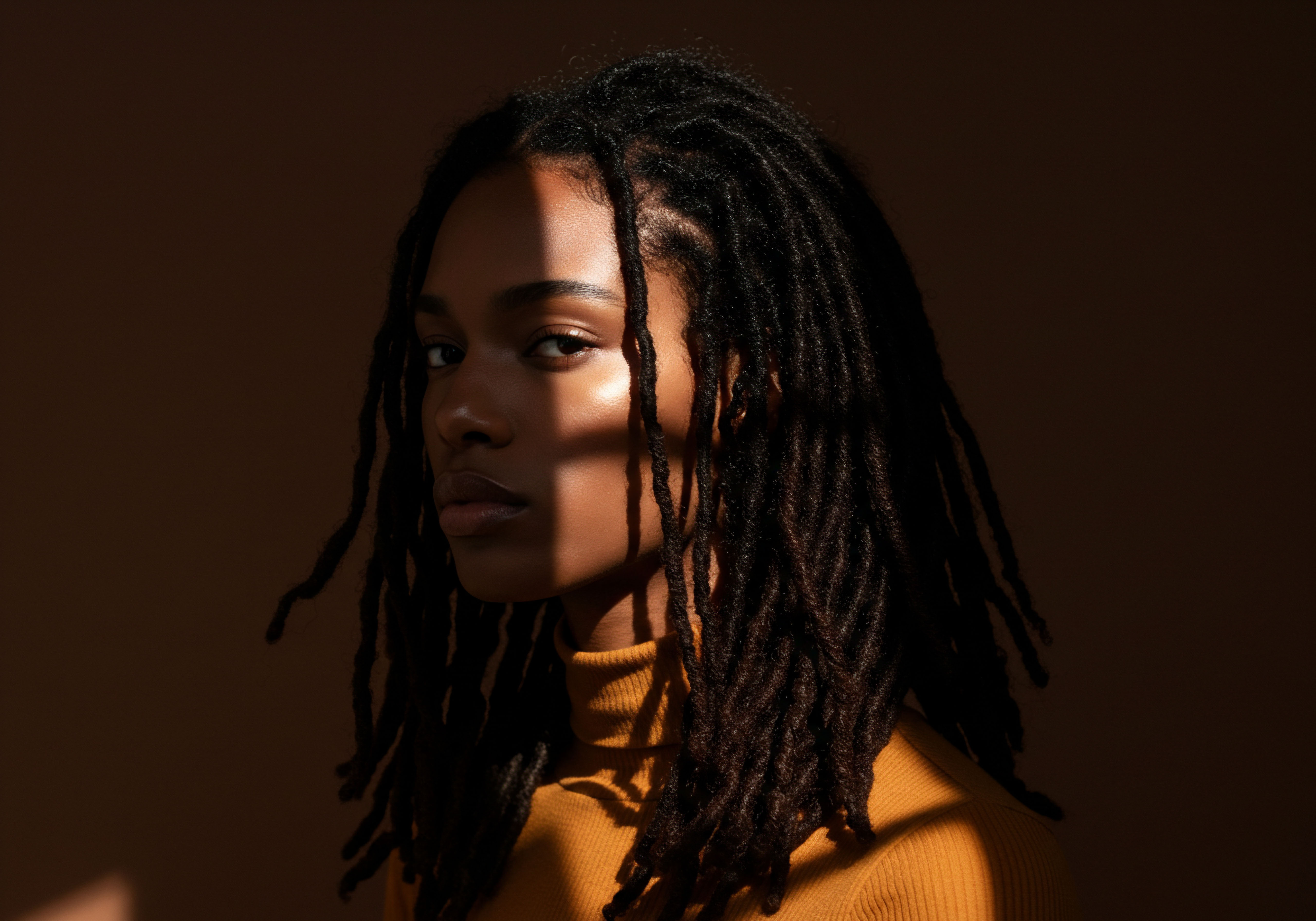
Roots
The quiet dance of water within a single strand of hair, a seemingly simple occurrence, holds profound implications for how that strand behaves, particularly concerning its interaction with electrical currents. From the gentle sway of a healthy curl to the sudden, surprising cling of static on a dry day, water’s presence dictates much of hair’s responsiveness to its surroundings. This elemental relationship between water and hair’s electrical flow is not merely a scientific curiosity; it forms the very foundation of understanding how textured hair lives, breathes, and responds to our care. We seek to peel back the layers of this intimate connection, revealing the hidden mechanisms that govern hair’s electrical life, starting from its deepest structural heart.
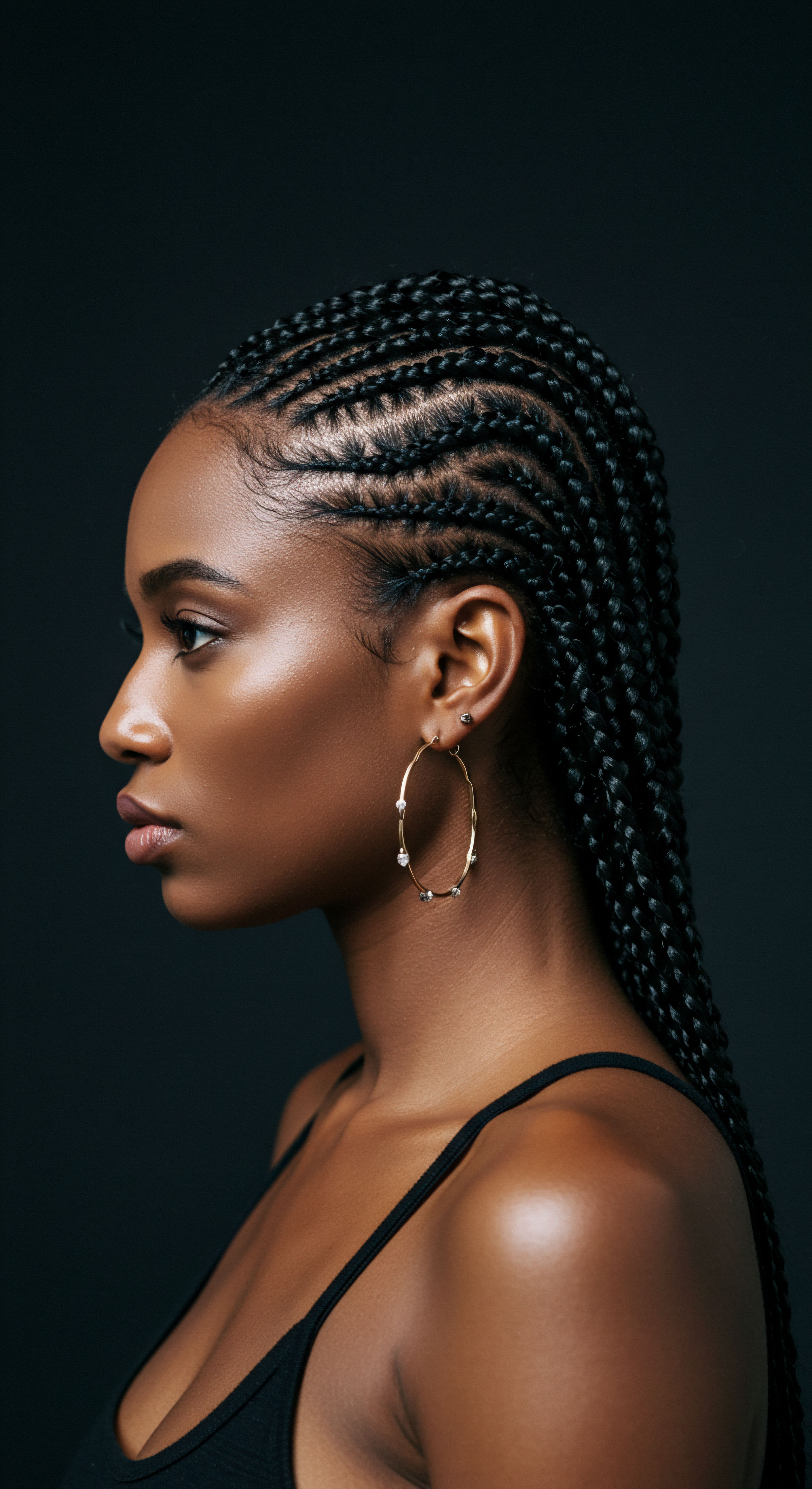
The Architecture of Hair and Water’s Place
A strand of hair, often perceived as a singular entity, is a marvel of biological engineering. At its core lies the Medulla, a soft, sometimes absent, central region. Surrounding this is the Cortex, the primary bulk of the hair fiber, composed of highly organized keratin proteins. These proteins, wound into α-helical structures, give hair its strength and elasticity.
The outermost layer, the Cuticle, consists of overlapping, scale-like cells that protect the inner cortex. Water, in its various forms, finds its way into all these layers, but its interaction is most significant within the protein-rich cortex and the surface of the cuticle.
Water molecules are small and highly polar, meaning they possess a slight positive charge on one side and a slight negative charge on the other. This polarity allows them to form Hydrogen Bonds with the polar groups found on the keratin proteins within the hair fiber. These bonds are not static; they are constantly forming and breaking, creating a dynamic environment within the hair.
The amount of water present influences the hair’s internal structure, affecting the spacing between keratin chains and the mobility of molecular segments. This internal fluidity, dictated by hydration, directly impacts how electrical charges can navigate the hair’s pathways.
Water’s presence within hair fibers profoundly shapes their electrical characteristics, transforming them from insulators to conductors depending on hydration levels.
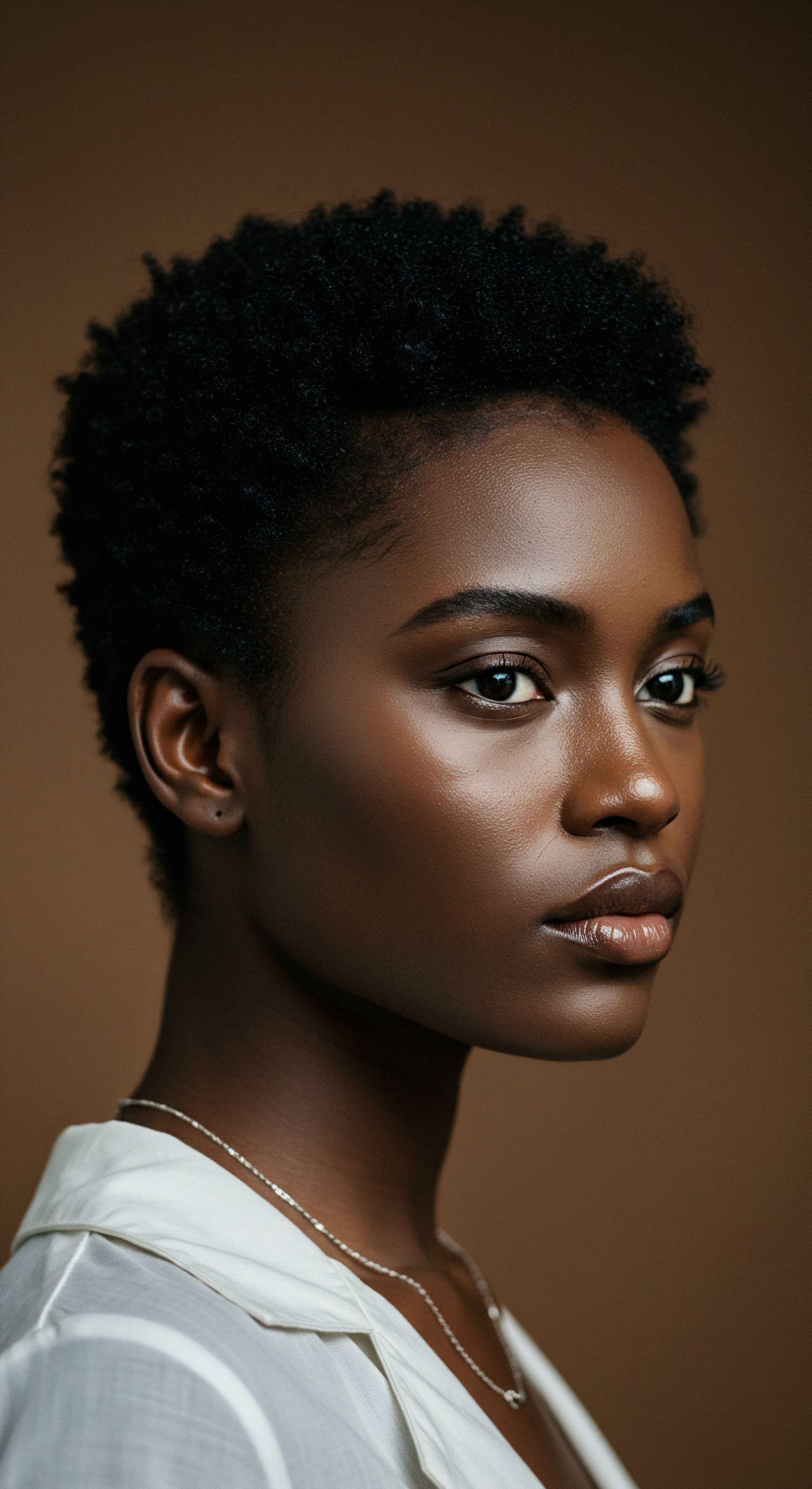
Hair as a Material ❉ Insulator or Conductor?
Dry hair, in its natural state, is generally considered an electrical insulator. This means it resists the flow of electrical current. The tightly packed, relatively immobile keratin proteins and the minimal presence of free water molecules do not offer easy pathways for charge carriers (like ions or electrons) to move. However, as hair absorbs water, its electrical properties undergo a remarkable shift.
The dielectric properties of hair, which describe how an electrical field affects a material, are highly dependent on moisture levels. Research indicates that as relative humidity increases, the Direct Current Conductance of human hair rises significantly. For instance, a study found that the conductance of human hair can increase by more than 104 times when relative humidity changes from 31% to 85%. This dramatic change signals that water transforms hair from a resistive barrier into a more conductive medium.
This phenomenon is rooted in how water interacts with the hair’s molecular structure. Water molecules, particularly those that are not tightly bound to the keratin, can act as mobile charge carriers or facilitate the movement of ions already present within the hair fiber. The internal environment of the hair becomes more amenable to electrical flow as water molecules create pathways for these charges to travel.
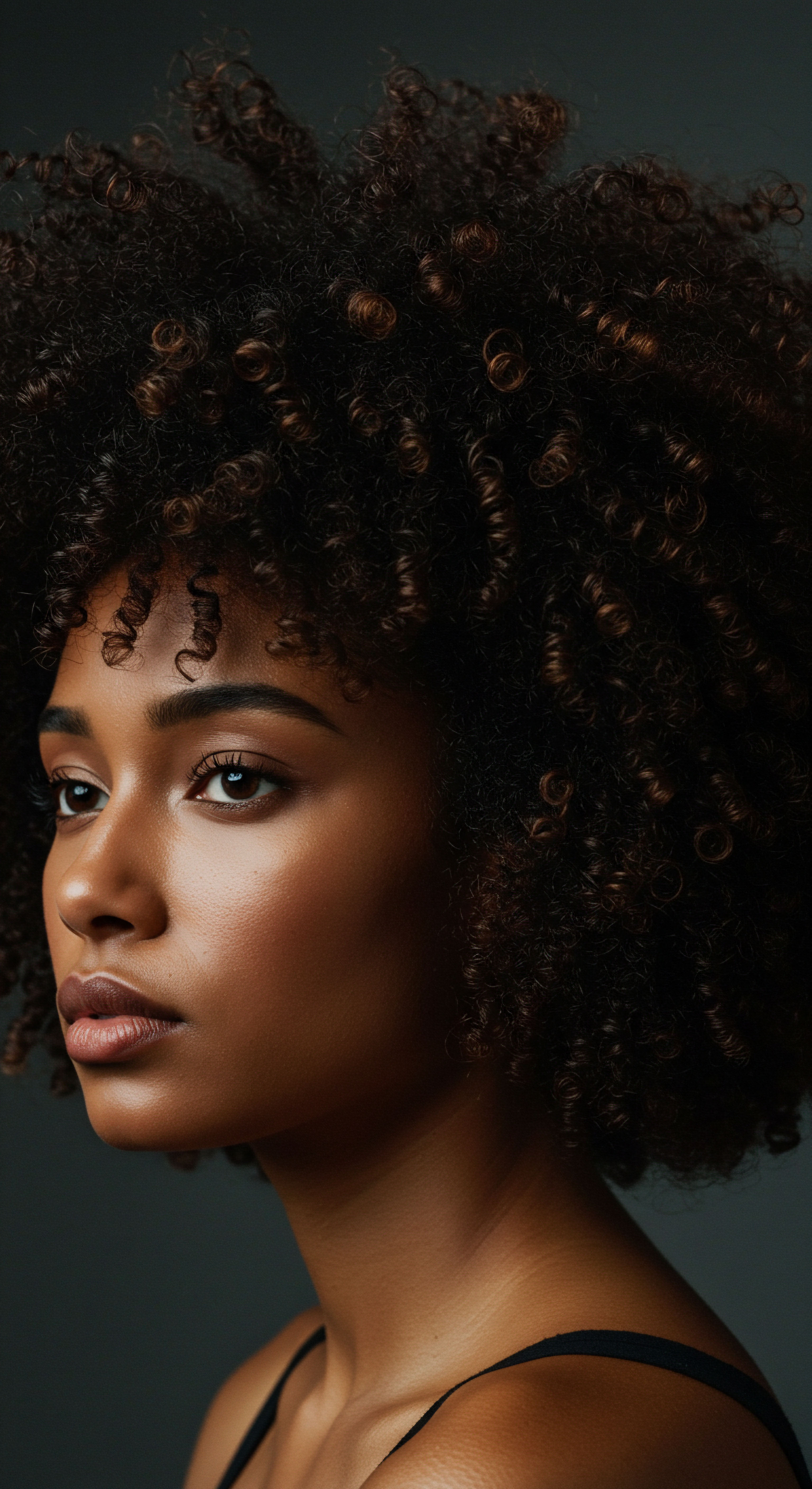
How Water Facilitates Electrical Flow
The mechanism by which water alters electrical flow in hair is multi-layered. Firstly, water itself, especially when containing dissolved ions (from products, environmental exposure, or natural hair composition), provides mobile charge carriers. Secondly, water molecules, through their ability to form and break hydrogen bonds, increase the molecular mobility within the keratin structure. This increased flexibility allows for easier movement of any existing ions or charged groups along the protein chains.
- Hydronium Ions ❉ Water can dissociate into hydronium (H3O+) and hydroxide (OH-) ions. These ions, especially hydronium ions, can “hop” from one water molecule to another, or along a chain of water molecules, creating a proton conduction pathway.
- Bound Water Mobility ❉ Even water molecules closely associated with keratin proteins contribute. As more water is absorbed, the “loosely bound” water fraction increases, offering more pathways for ionic movement.
- Swelling and Structural Changes ❉ Water causes hair fibers to swell. This swelling can open up spaces within the protein matrix, allowing for greater ionic mobility and reducing the electrical resistance of the fiber.
The journey of understanding hair’s electrical life begins with acknowledging water’s quiet yet powerful role in shaping its very conductive nature. This foundational knowledge allows us to approach hair care with a deeper respect for its delicate balance.
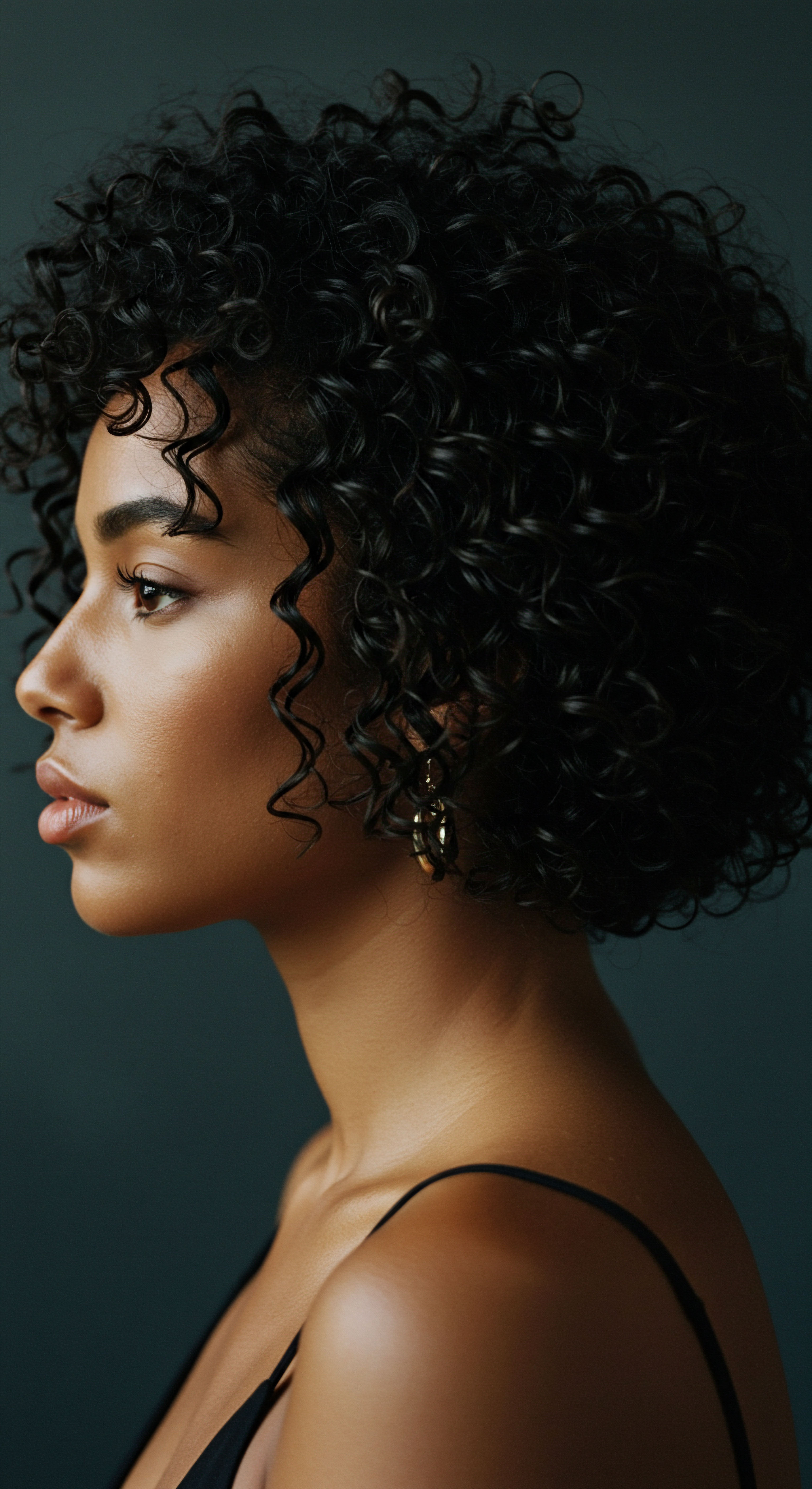
Ritual
Our daily rituals with textured hair are often intuitive, guided by touch and observation, yet beneath the surface, they are deeply intertwined with the hair’s electrical responses to water. Consider the serene feeling of damp, pliable strands, easy to detangle, or the frustration of dry, flyaway hair that seems to defy gravity. These common experiences are not coincidental; they are direct manifestations of how water content influences the electrical flow within hair fibers, shaping our interactions with our coils and curls. This section steps into the practical wisdom of hair care, exploring how moisture, in our hands, transforms hair’s electrical state and, by extension, its behavior.
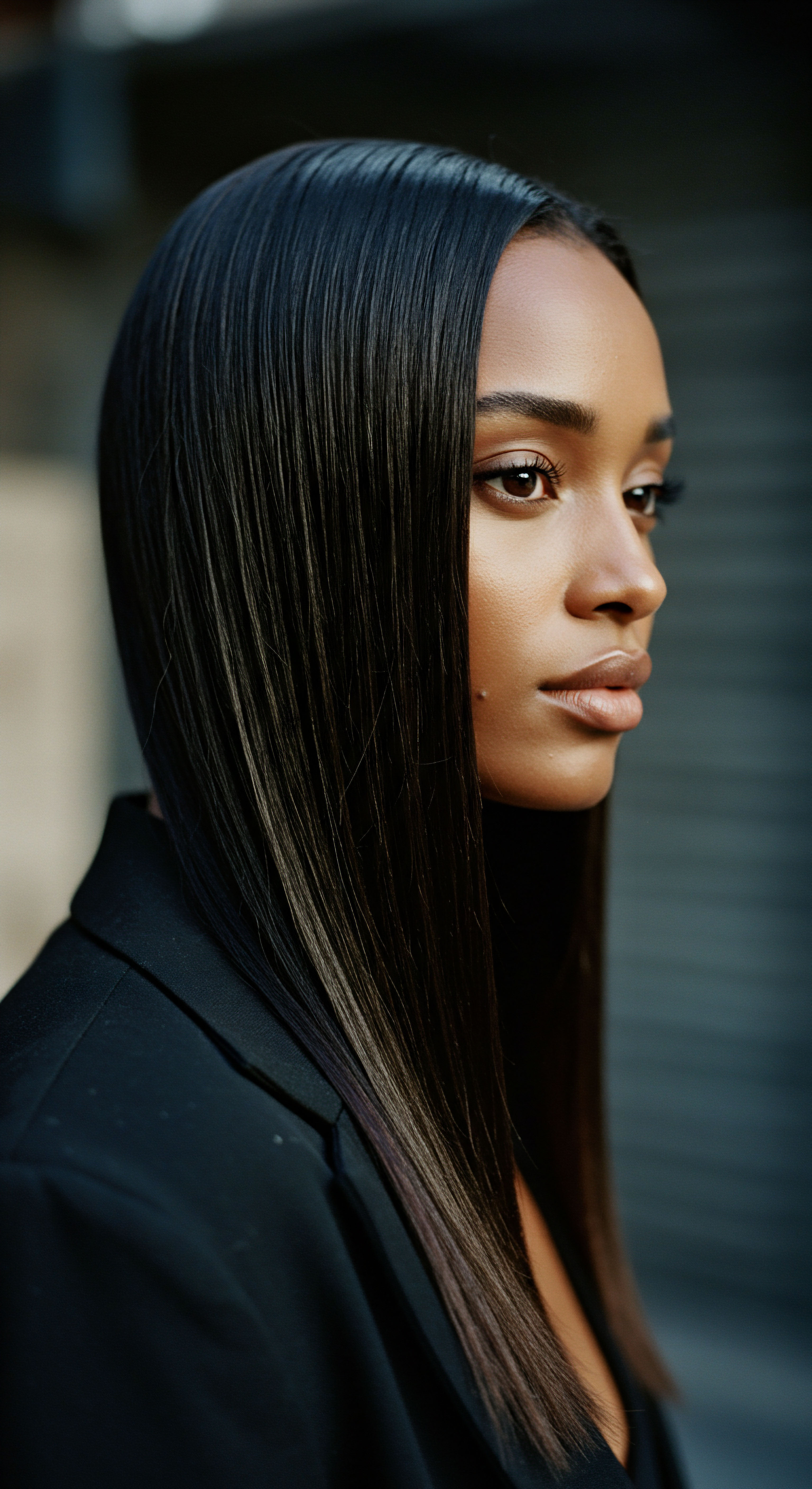
Water’s Daily Dance with Static
One of the most noticeable impacts of water content on hair’s electrical properties is the phenomenon of static electricity. When hair is dry, especially in low humidity environments like heated indoor spaces during colder months, it readily acquires an electrical charge through friction – a process known as the Triboelectric Effect. This happens when hair rubs against fabrics like scarves, hats, or even our clothes, causing electrons to transfer. Hair strands, having gained or lost electrons, then repel each other due to like charges, leading to the familiar “flyaway” appearance.
The presence of water significantly mitigates this static charge. Water molecules, being polar, can dissipate the electrical charge by providing a conductive pathway for the excess electrons to move away from the hair fiber. They act as a natural conductor, allowing the charge to equalize with the surrounding air or other surfaces.
This is why a light mist of water or a water-based leave-in conditioner can instantly calm static-prone hair. The higher the water content, the more effectively hair conducts and dissipates static charges.
Everyday hair rituals, from detangling to styling, subtly influence hair’s electrical state through the mindful management of its water content.
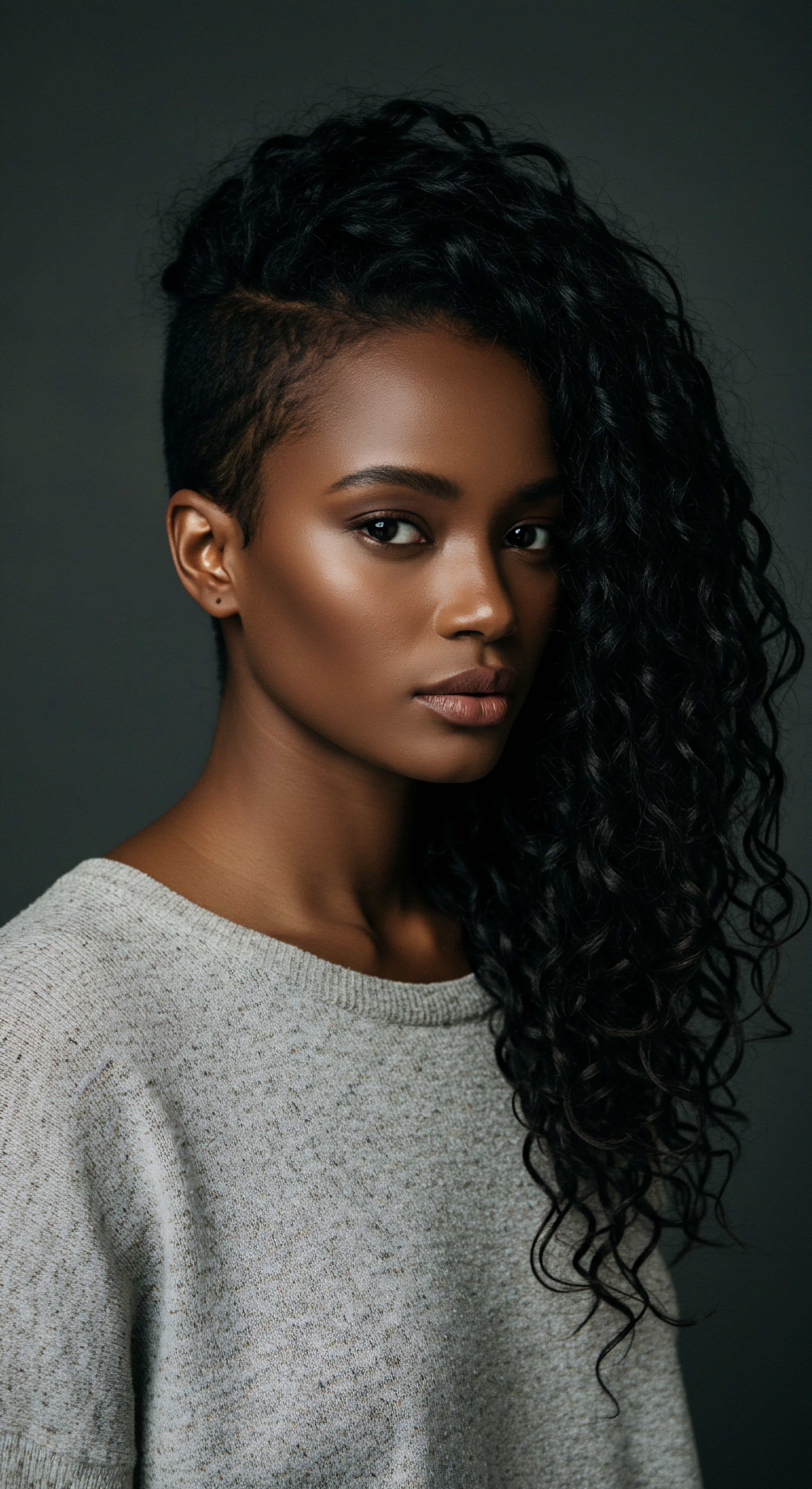
Product Interactions and Electrical Balance
The products we choose also play a critical role in managing hair’s water content and, consequently, its electrical flow. Many hair care formulations are designed with this delicate balance in mind:
- Humectants ❉ Ingredients like glycerin or hyaluronic acid draw moisture from the air into the hair. This increased hydration directly boosts the hair’s electrical conductivity, helping to reduce static and make hair more pliable.
- Cationic Conditioners ❉ Conditioners often contain positively charged (cationic) compounds that bind to the negatively charged surface of untreated hair. This binding neutralizes some of the hair’s natural negative charge, which can reduce static and smooth the cuticle. When hair is properly hydrated, these cationic ingredients can distribute more evenly, further optimizing the hair’s surface charge.
- Oils and Sealants ❉ While not directly adding water, oils and sealants help to lock in the existing moisture within the hair fiber, preventing rapid dehydration. By maintaining an optimal water content, these products indirectly help sustain the hair’s natural electrical balance and reduce the likelihood of static.
Consider the impact of hard water, which contains high levels of positively charged mineral ions like calcium and magnesium. These ions can deposit on the hair surface, potentially altering its natural negative charge and affecting how other conditioning agents bind. The interaction of these ions with the hair’s surface charge, particularly on damaged hair with more negatively charged sites, can impact the hair’s feel and its electrical responsiveness.
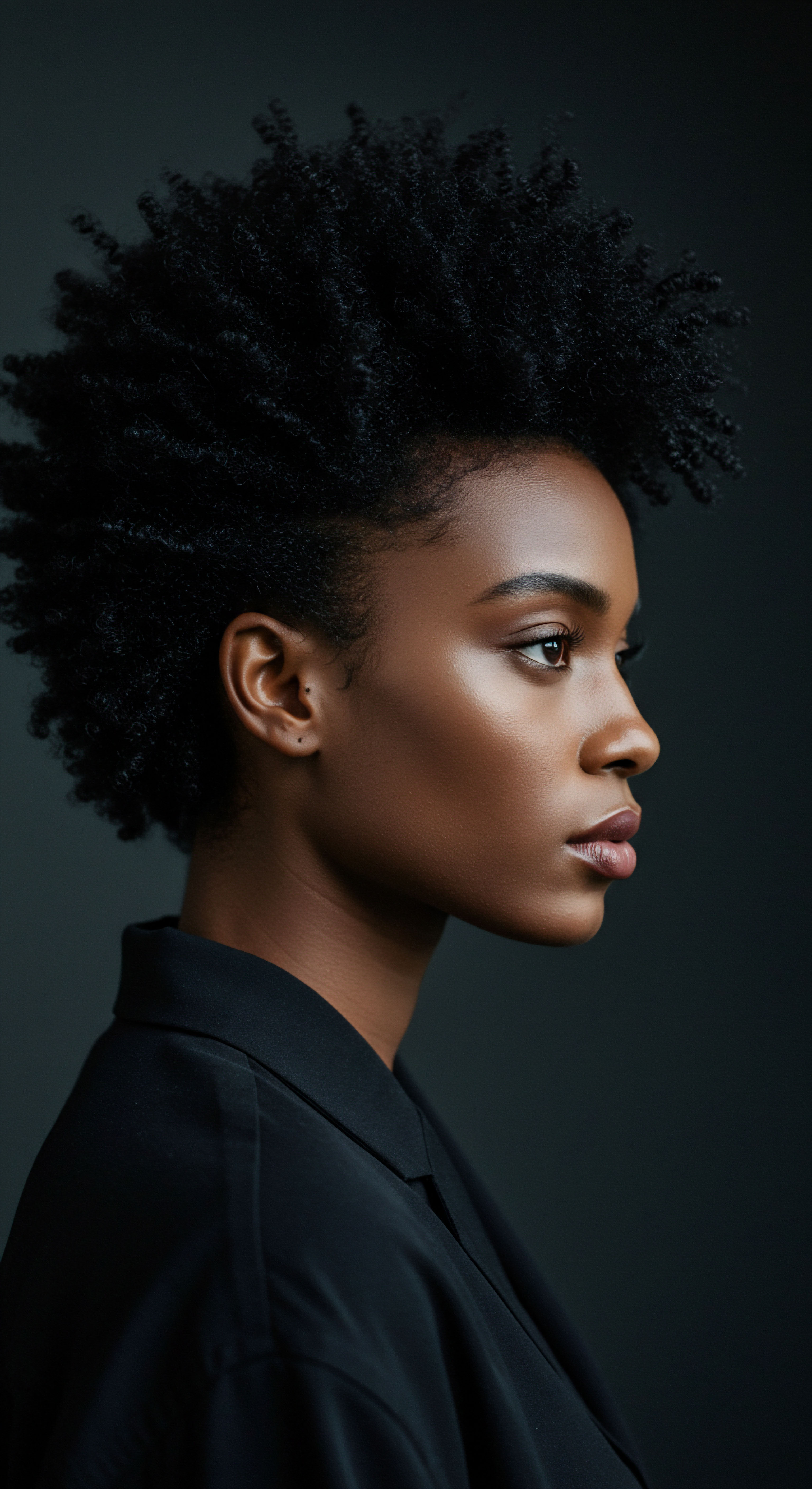
Styling Tools and Electrical Dynamics
Heat styling tools, such as blow dryers and flat irons, drastically alter the water content within hair fibers, with immediate consequences for electrical flow. As heat evaporates water, hair becomes drier and more susceptible to static and friction. This loss of moisture diminishes hair’s conductivity, allowing electrical charges to accumulate more readily.
Some modern styling tools, like ionic blow dryers, aim to counteract this. These dryers release negative ions, which are believed to neutralize the positive ions that can build up on hair during drying, thereby reducing static and helping to seal the cuticle. This technological approach directly addresses the electrical imbalance created by rapid water removal, aiming to restore a smoother, less charged state.
Our rituals, whether simple detangling or elaborate styling, are quiet conversations with our hair’s electrical nature. Understanding water’s role in this conversation allows us to approach hair care with greater intention, supporting the delicate electrical equilibrium that keeps our strands vibrant and cooperative.
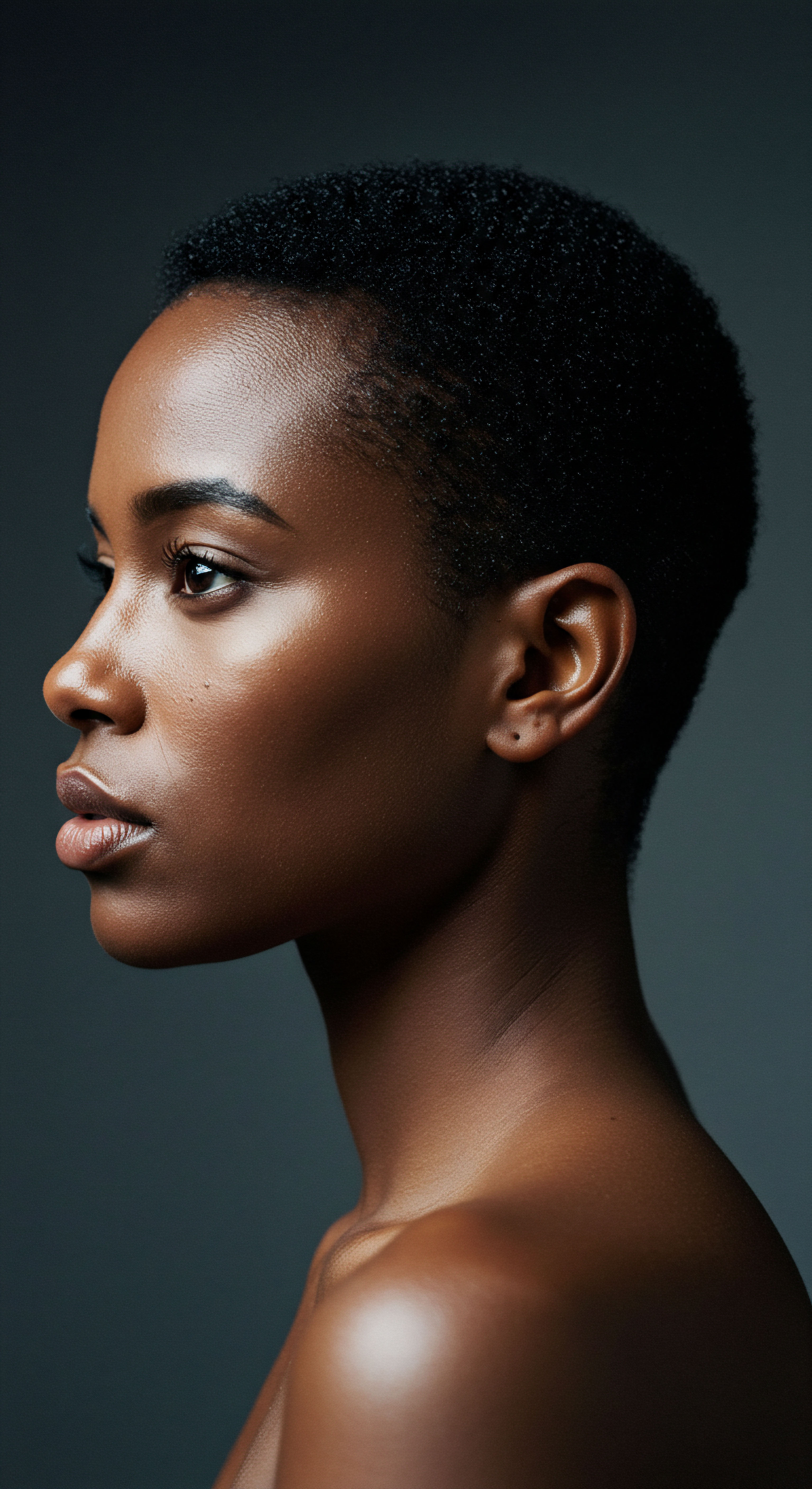
Relay
Moving beyond the visible effects of water on hair’s electrical flow, we uncover a more complex, almost unseen dialogue occurring at the molecular level. This is where science, culture, and subtle details converge, inviting a deeper consideration of hair as a living, electrically responsive entity. The ways water influences electrical flow within hair fibers extend far beyond static, reaching into the very core of its structural integrity and even its potential as a biosensor. This exploration delves into the intricate mechanisms and surprising data points that reveal hair’s profound electrical sensitivity to its hydration state.
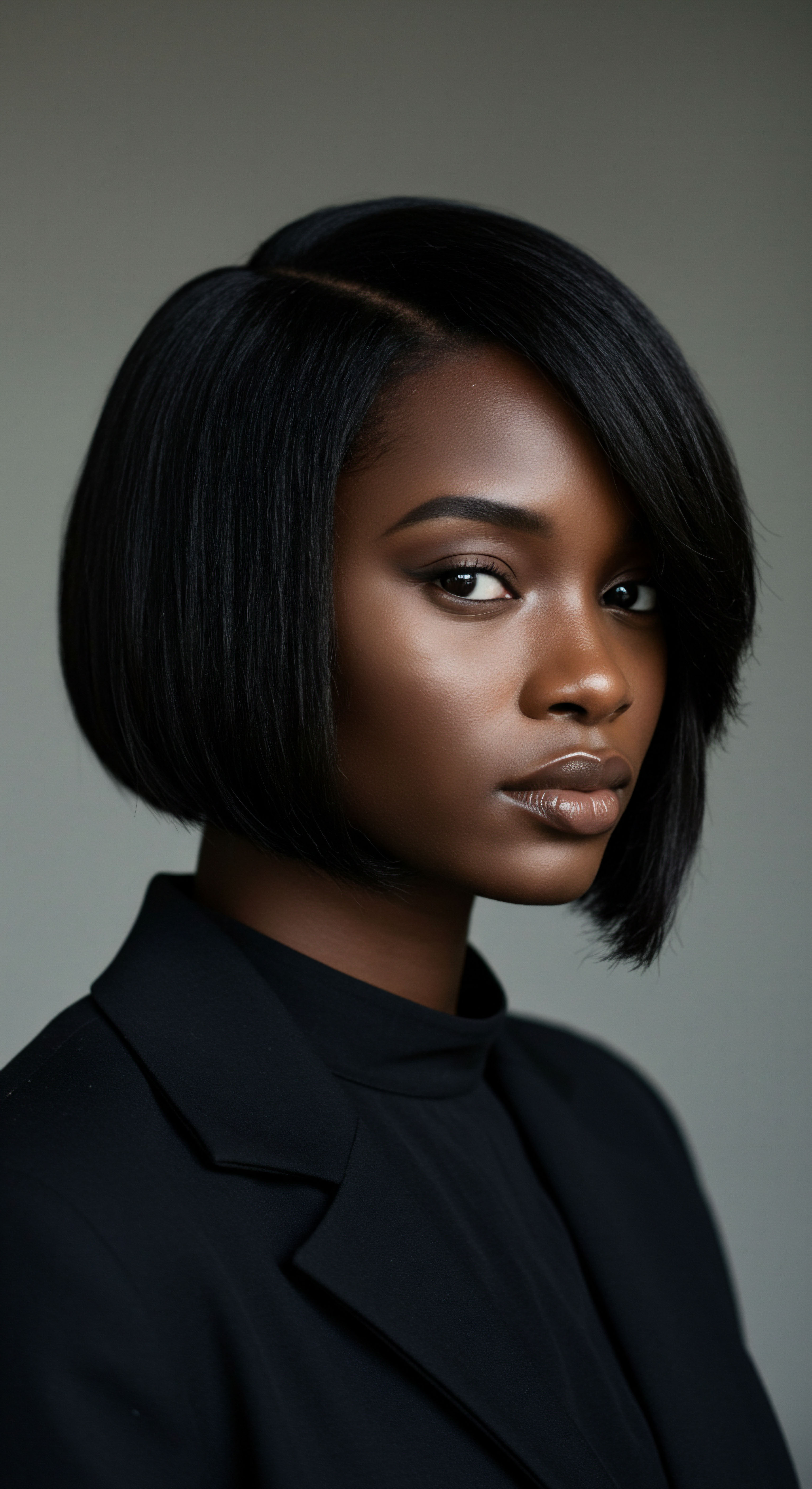
What Are the Mechanisms of Electrical Conduction in Hair?
The electrical conductivity of hair, particularly at varying hydration levels, is a subject of ongoing scientific inquiry. While dry hair acts as an insulator, the presence of water dramatically changes this property. The primary mechanisms of electrical conduction in hydrated keratin fibers are thought to involve both Ionic Transport and Proton Hopping.
Ionic transport refers to the movement of charged ions (like H+, OH-, or other dissolved salts) through the water pathways within the hair fiber. As water content increases, these pathways become more interconnected and expansive, allowing ions to move with greater freedom. Proton hopping, a more specialized form of conduction, involves the rapid transfer of protons along a chain of hydrogen-bonded water molecules or polar groups within the keratin structure. This mechanism is particularly significant in biological systems where water plays a structural and functional role.
A study exploring the electrical properties of human hair found that its direct current conductance is substantially dependent on moisture level. The research highlighted that at high moisture levels, conductance becomes almost frequency-independent below 1 kHz. Furthermore, it revealed that the increase in conductance can continue for several days after the hair fiber has reached equilibrium with ambient relative humidity, suggesting a complex, slow process of water absorption contributing to electrical pathways. This implies that the internal arrangement of water molecules and their interaction with keratin proteins are not instantaneous but evolve over time, continuously altering the hair’s electrical landscape.
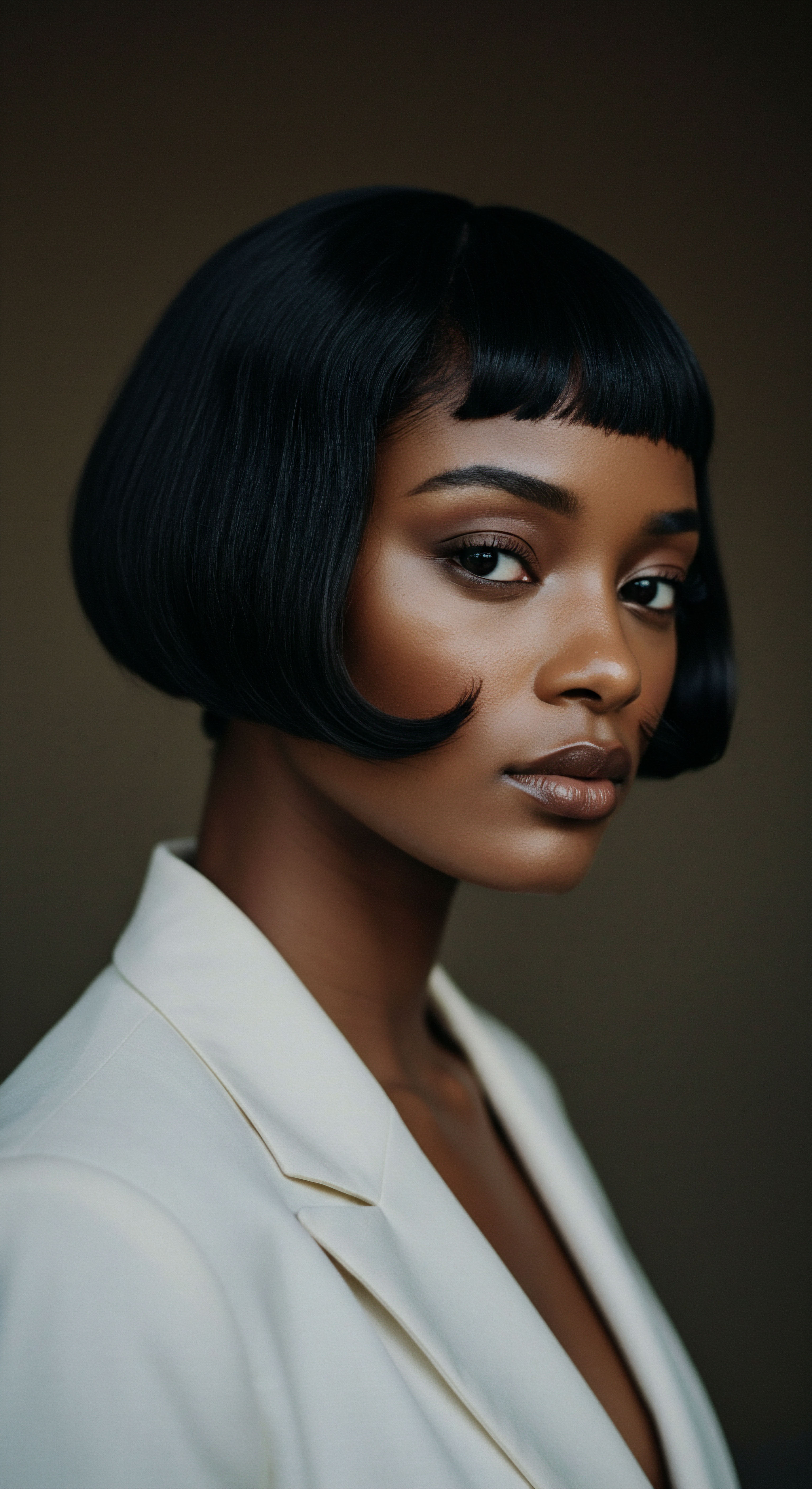
Can Hair Act as a Humidity Sensor?
The remarkable sensitivity of hair’s electrical properties to water content has led to its historical and contemporary use in humidity sensing. For centuries, hygrometers, instruments for measuring humidity, have utilized human or horse hair due to its characteristic length changes in response to moisture absorption. Beyond mechanical changes, hair’s electrical response offers another avenue for sensing.
Recent advancements demonstrate hair’s potential in sophisticated biosensing applications. Research has shown that naturally extracted Keratin, the primary protein in hair, can be used as an active ionic electrolyte in bendable micro-electrode arrays for monitoring humidity flow. These devices capitalize on the reversible binding and extraction of water ions from the keratin layer, demonstrating a linear correlation between controlled humidity levels and the amperometric output signal. This application highlights hair’s inherent electrical responsiveness to its environment, transforming a seemingly simple biological material into a functional component of advanced technology.
Hydration State Dry Hair (Low Humidity) |
Primary Electrical Characteristic High Resistance (Insulator) |
Mechanism Limited mobile charge carriers; tightly bound keratin structure restricts ion movement. |
Hydration State Damp Hair (Moderate Humidity) |
Primary Electrical Characteristic Increased Conductance |
Mechanism Water molecules provide some mobile ions and facilitate proton hopping along protein chains. |
Hydration State Wet Hair (High Humidity) |
Primary Electrical Characteristic Significantly Increased Conductance |
Mechanism Abundant water creates extensive pathways for ionic transport and enhanced proton hopping. |
Hydration State Hair's electrical behavior shifts dramatically with moisture, enabling varied responses. |
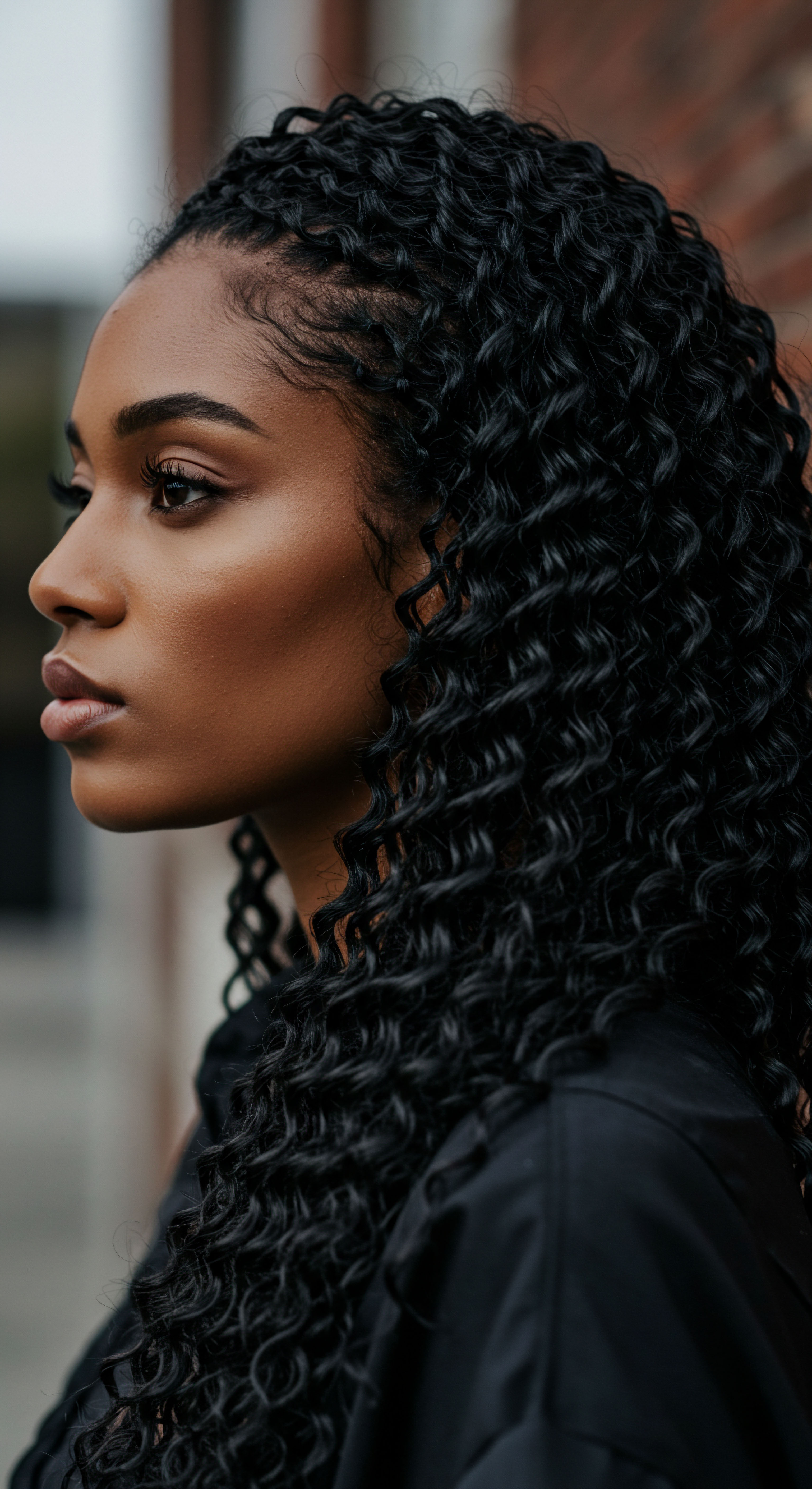
How Do Environmental Factors Influence Hair’s Electrical Balance?
Beyond direct water content, environmental elements play a role in the hair’s electrical state. Temperature, for instance, affects the mobility of water molecules and ions within the hair fiber. Higher temperatures can increase molecular motion, potentially enhancing conductivity, especially when coupled with adequate hydration. A study on the universality of AC conductance in human hair noted that conductance increases with both relative humidity and temperature, suggesting a combined effect on ionic and molecular mobility.
The chemical composition of water, such as the presence of metal ions from hard water, can also influence hair’s electrical properties. These ions can deposit on the hair surface and within the fiber, interacting with negatively charged sites on keratin. This interaction can alter the hair’s overall surface charge and how it responds to external electrical stimuli or even other hair care products. For example, some metal ions are known to bind to hair, potentially impacting its ability to absorb and release moisture, which then has a secondary effect on its electrical behavior.
The intricate relationship between water content and electrical flow in hair is a testament to the sophistication of this biological material. It reminds us that hair is not merely a static adornment but a dynamic, electrically sensitive part of our being, constantly interacting with its environment in ways both seen and unseen.
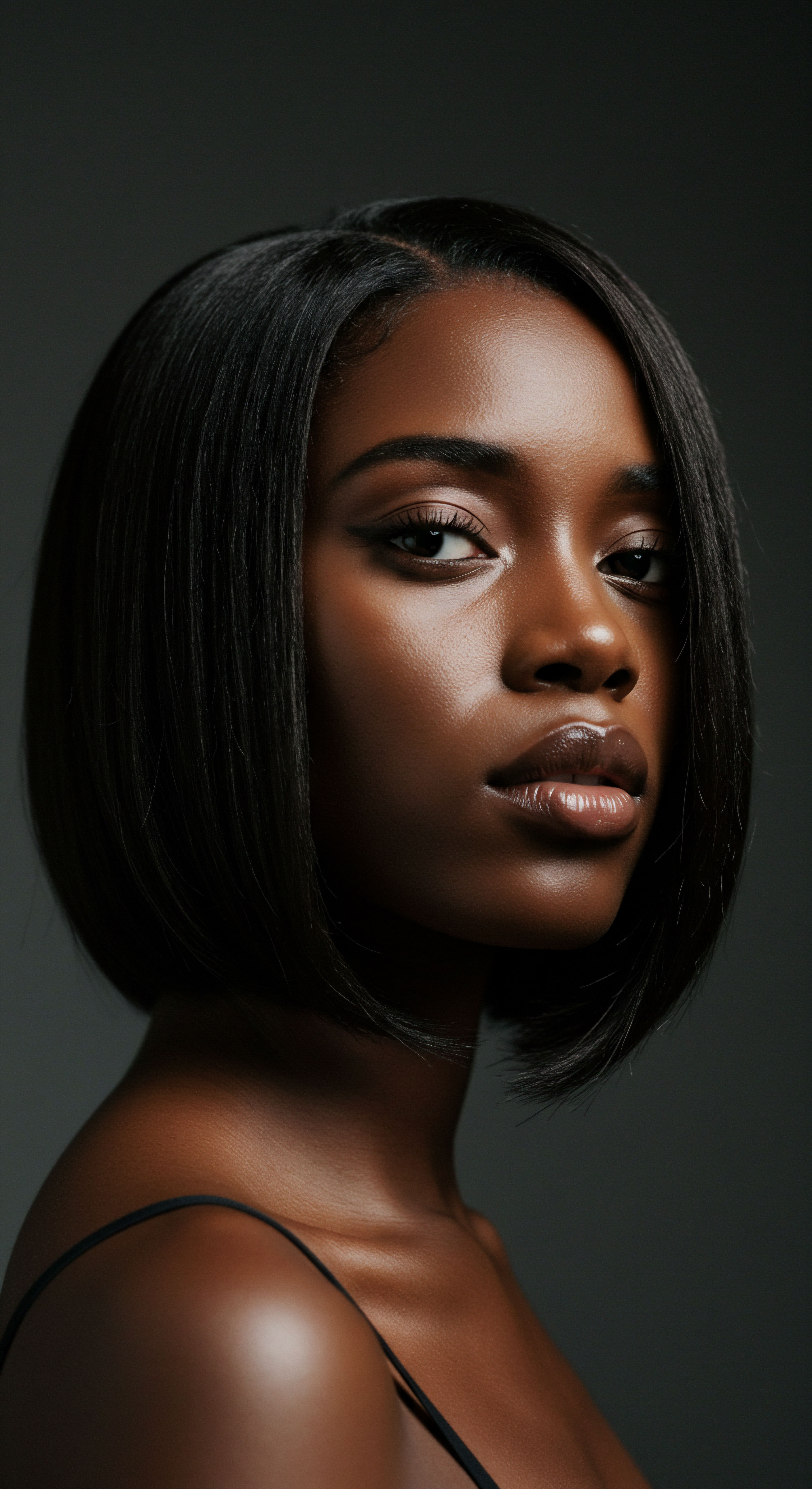
Reflection
The subtle dance of water within each hair fiber orchestrates a silent symphony of electrical responses, shaping how our coils and curls interact with the world around them. From the soft resistance of dry strands to the lively pliability of those touched by moisture, hair’s electrical nature is a testament to its living, responsive quality. This deeper understanding invites us to approach hair care not as a series of rigid rules, but as an intuitive engagement with a dynamic system, recognizing that every drop of water, every environmental shift, holds a unique influence over its electrical spirit. We are invited to observe, to listen, and to care for our hair with a reverence for its intricate, electrically charged life.
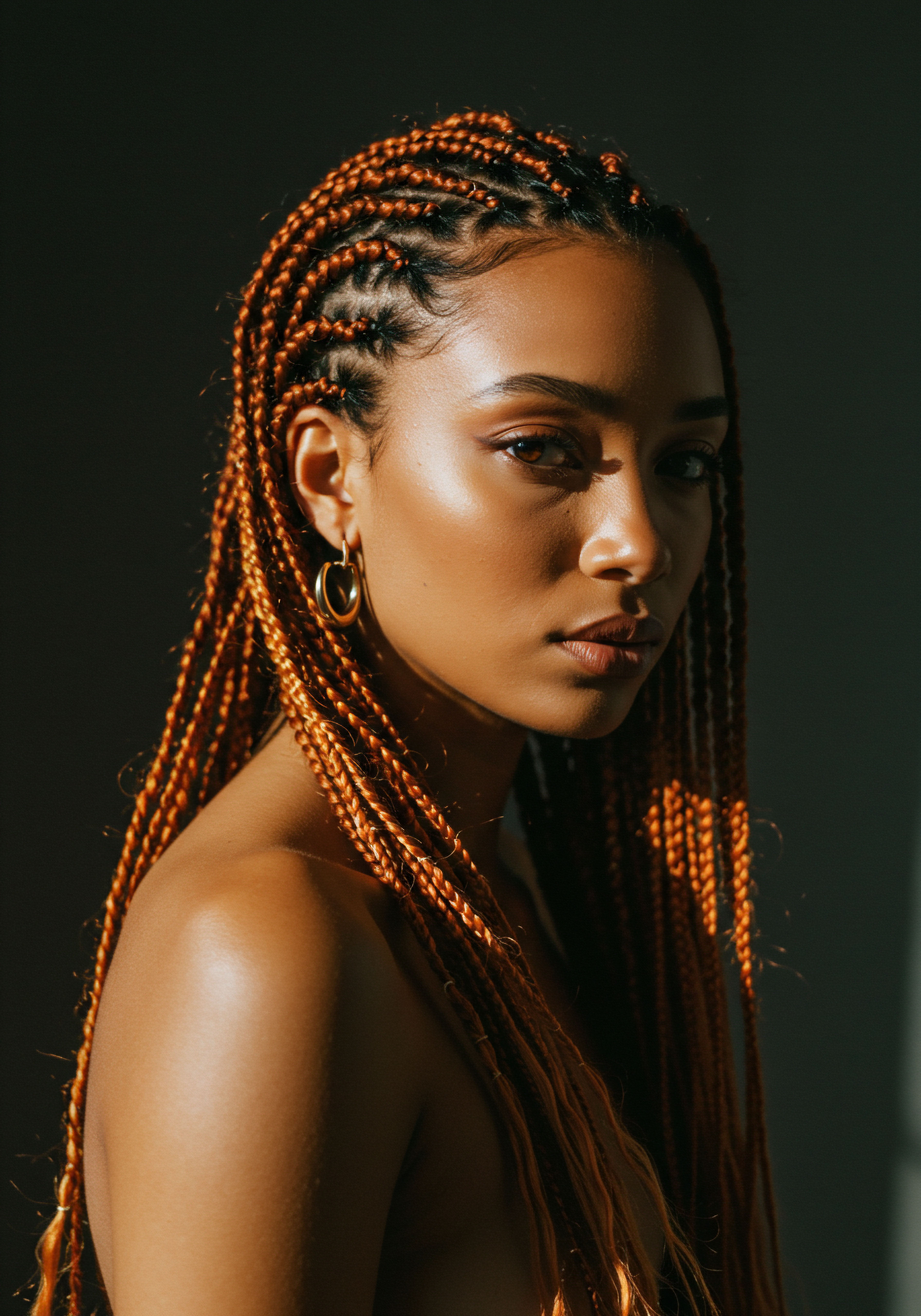
References
- Martinsen, Ø. G. Grimnes, S. & Kongshaug, E. (1997). Dielectric properties of some keratinised tissues. Part 2 ❉ Human hair. Medical & Biological Engineering & Computing, 35(2), 177-180.
- Sobottka, G. & Weber, A. (2006). Computing Static Electricity on Human Hair. Eurographics Association.
- Nath, S. & Singh, A. (2019). Harvesting electricity from human hair. Journal of Renewable and Sustainable Energy, 11(4), 044701.
- Natali, M. Nardecchia, S. & Papi, M. (2019). Engineering of keratin functionality for the realization of bendable all-biopolymeric micro-electrode array as humidity sensor. Biosensors and Bioelectronics, 143, 111624.
- Algie, J. E. (1958). The Effect of Strain on the Electrical Resistance of Keratin. Textile Research Journal, 28(12), 1007-1011.
- Martinsen, Ø. G. & Grimnes, S. (2016). Universality of AC conductance in human hair. Medical & Biological Engineering & Computing, 54(1), 289-294.
- Xiao, P. Ciortea, L. I. Bontozoglou, C. & Imhof, R. E. (2012). Hair Water Content and Water Holding Capacity Measurements. Cosmetics and Toiletries, 127(7), 470-475.
- Tiwari, P. et al. (2020). Piezoelectric Biopolymers ❉ Advancements in Energy Harvesting and Biomedical Applications. Polymers, 12(11), 2608.
- Osorio, F. & Tosti, A. (2011). Hair weathering. Part 1. Hair structure and pathogenesis. Cosmetic Dermatology, 24(11), 533-538.
- Martinsen, Ø. G. Grimnes, S. & Nilsen, S. (2008). Water sorption and electrical properties of a human nail. Skin Research and Technology, 14(2), 142-146.